Research Projects
The Electrical Engineering Department at UTRGV has active research programs in biomedical microdevices, semiconductor devices and modeling, VLSI circuits, electromagnetics and antennas, high-speed and wireless networking, signal and image processing, controls and robotics, and power systems.
Biomedical Microdevices
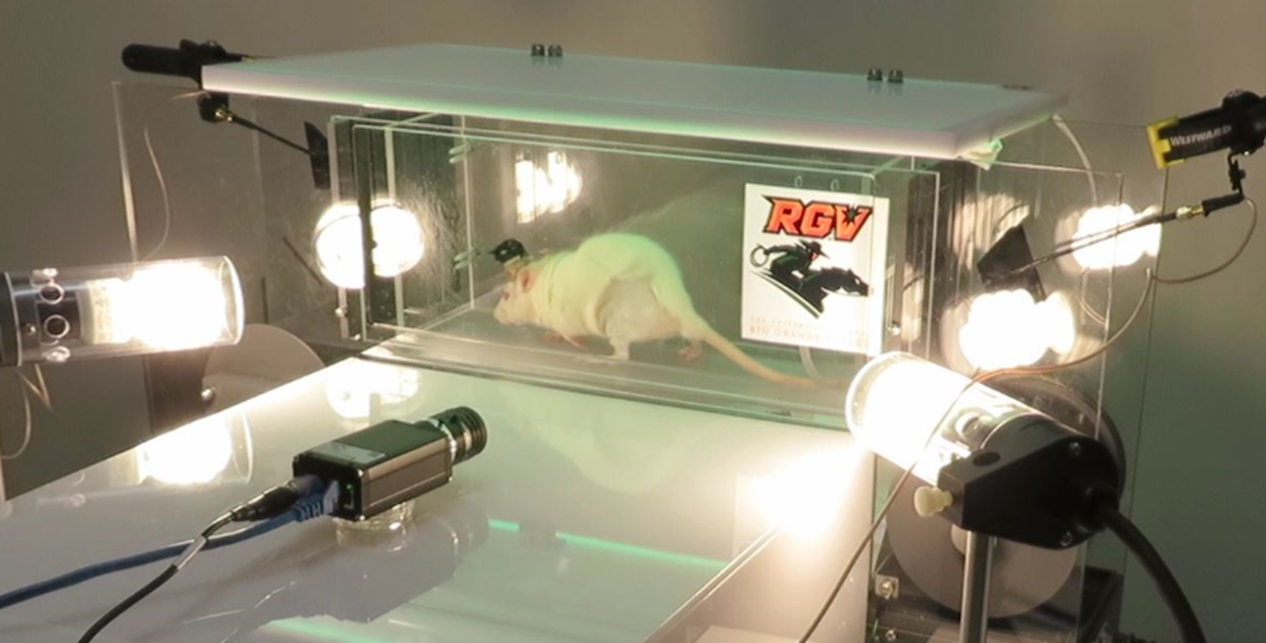
Dr. Paul Choi's research group has developed a neural interface with the peripheral nervous system to provide a direct communication pathway between peripheral nerves and external devices such as prosthetic limbs. This research has pursued a regenerated peripheral nervous system which controls reinnervated muscles and interprets neurological signals matching specific behavior patterns of animals. The acquired bioelectrical signals can be used for the interpretation of mind which will be used to maneuver prosthetic limbs. Rodent animal studies have been performed to evaluate implanted peripheral nerve interfaces using KinemaScan gait analysis system. Both TBSI wireless system and TDT high-density system have been used to stimulate and record electrophysiological signals from the brain and the peripheral nerves. This project has been supported by the UT System STARS program and the Army Research Office.
Networking, Switching/Routing, Computer Security
Dr. Sanjeev Kumar’s research encompasses a broad spectrum of theoretical and applied research activities, involving all types of networks – wireless and wireline data networks, sensor networks, and high performance broadband networks. Significant emphasis is placed on design and analysis of protocols, networking architecture, configurations and measurements for performance efficiency, security, and survivability of wireless and wireline networks. Dr. Kumar is the founder of NSF funded Networking Research Lab (NRL) which houses leading-edge networking equipment. The NRL research activities have played a major role in design & evaluation of new architecture and protocols of next generation networks, and secure network systems. NRL is uniquely positioned to provide graduate research students hands-on experience which is highly sought after in telecom, data and wireless Industry. Dr. Kumar’s research group is actively solving research problems that result in significant practical impact.
Sputtering System for Device and Material Research(SDMR) Laboratory
A recent NSF MRI grant has supported the acquisition of a sputtering system at Hispanic Gateway Institutions. The sputtering system supports ongoing multidisciplinary research and provide student training in semiconductor devices and materials research at both the Edinburg and Brownsville Campuses.
Wide Bandgap Semiconductors
Research is being conducted by Dr. Hasina Huq for a high-performance building block for high frequency and high temperature applications that combine lower costs with improved performance and manufacturability. Researchers have focused their attention on new semiconductor materials for use in device technology to address system improvements. Of the contenders, silicon carbide (SiC), gallium nitride (GaN), and diamond are emerging as the front-runners. Temperature Dependent Analytical Modeling, Simulation and Characterizations of devices are being investigated. The models developed in this research will not only help the wide bandgap device researchers in the device behavioral study but will also provide valuable information for circuit designers.
Device Modeling & Measurements
UTRGV has facilities for high-frequency characterization of devices. A 50 GHz high frequency time domain measurement system funded by NSF has been set up and InGaAs/AlGaAs transistors have been measured and correlated to S parameter measurements. Additional GaN high pulse power time domain measurement is progress. Two dimensional Monte Carlo and SILVACO PDF simulation of HEMT and MOSFET devices down to nanoscale dimensions has been investigated including quantum effects using the quantum potential. Further work in the nanoscale range is in progress.
- Development of a high frequency time domain microwave measurement capability correlated with frequency domain measurement.
- Extension of the time domain microwave measurement method to high power GaN semiconductors.
- Simulation of microwave solid state devices using Monte Carlo simulation that includes quantum effects.
- Simulation of nanoscale solid state devices to include two dimensional quantum effects.
- Simulation of microwave high power GaN solid state devices using SILVACO software.
- Simulation and parameter extraction of BSIM4 model parameters related to quantum effects using SILVACO simulation and ICCAP parameter extraction.
VLSI, Asynchronous Digital Design, Low-Power IC Design
Dr. Weidong Kuang’s research interests include VLSI design, low power digital ICs, asynchronous circuits and deep submicron VLSI reliability issues.
- Low Power Design. Power consumption is a critical constraint influencing the design and operation of a wide variety of computing and communication systems. Power-related metrics have become important for different reasons, such as reducing cooling and packaging costs, prolonging battery life, and increasing reliability of the system. Asynchronous circuits have promising low power performance. And double-edge triggered flip-flops will save power compared to traditional flip-flops which are single-edge triggered.
- Soft Error Correction. Reliability is one of the major concerns for advanced semi-conductor manufacturers. Among these concerns are soft errors caused by alpha particles, terrestrial cosmic rays and thermal neutrons that spontaneously flip bits of stored data in a memory or logic chip, resulting in system crashes and network failure. Memories have been successfully protected from soft errors by error correction codes. There is no effective way to improve logic circuit soft error reliability. Asynchronous circuits demonstrate different behaviors from synchronous circuits in the presence of soft errors. The dual-rail encoding and quasi delay insensitivity in asynchronous circuits make it possible to effectively detect and correct soft errors.
Power Generation and Electromechanics
Dr. Jaime Ramos is conducting research on a number of projects in the area of power systems.
- Power Systems Harmonics. We are presently measuring harmonic currents in the Campus’ main low voltage circuits. We have designed low voltage harmonics filters, and we are setting up a filter, and we expect to see soon a third harmonic suppression. We plan to use active filters. We are designing analog to digital converters to digitalize the power frequency waveforms.
- Power Electronics. We recently submitted a proposal for opening an electric Machinery and Power Electronics Laboratory. This Lab will complement the motors, drives, and other power equipment already present in the Sophomore Lab. This Lab will let us do research in the area of efficient use of electrical energy.
- Dielectric strength of materials. Dr. Ramos recently co-authored a proposal, together with faculty members of Mechanical & Manufacturing Engineering to set up a Nondestructive Evaluation Laboratory for Ceramic Bearings. This project is an off-spring of the Nano Tech Group at UTRGV.
Radar, Antennas, and UWB Electromagnetics
Dr. Junfei Li's research interests include Radar Image Processing, Electromagnetic Inverse Scattering, Radar & Microwave Instrumentation, Radar Signal Processing and Imaging, Microwave Remote Sensing, Electromagnetic Inverse Scattering, Ultra-Wide-Band Radar. Dr. Heinrich Foltz works in the areas of antenna design, antenna theory, UWB electromagnetics and RF circuits. Some current projects are:
- Radar for Improvised Explosive Device Detection, in collaboration with UTSA, funded by the Air Force Force Protection Battle Lab
- Acquisition of Ultra-Wide-Band Measurement and Modeling Facilities for Multidisplinary Research, funded by the National Science Foundation
- Ultra-Wideband Microwave Imaging for Biomedical Applications, funded by the Texas Advanced Research Program
- High-Performance Ultra-Wideband Antennas, funded by the Army Research Office.
Modern communications, location, and sensing systems employing UWB waveforms require a thorough understanding of physical scattering mechanisms as well as antennas with excellent bandwidth characteristics combined with small size and high efficiency. Our research group works on development and characterization of new antenna designs; theoretical limits on wideband antenna performance; applications of broadband antennas in radar, communications, and medicine; and analysis of scattering through simulation and measurements.
Radar Imaging System Research
Dr. Jae Sok Son's research interests include Radar Signal & Image Processing, and Radar Motion Compensation. His research program is currently focused on the use of signal processing technology in synthetic radar image formation, enhancement, target recognition, motion estimation, and motion compensation. Although radar signal processing technology has been used for several decades to detect targets in all weather conditions, it has been successfully employed to only a limited extent in high resolution radar imagery. Therefore, multi-rate signal processing combined with maximally likelihood motion estimation can not only detect a target but form a high resolution image from a noisy radar data. The algorithm developed can be extended to the statistical signal processing, digital and voice communications.
- Speech and image compression, recognition and processing.
- Radar signal processing and target recognition.
- Digital and statistical signal processing implementation and application.
- Optimal filters and neural networks.
- Digital and voice communications
Wireless Networking
Dr. Jun Peng’s research interests are in computer communication networks. His most recent projects are on how to improve the medium utilization of distributed wireless packet networks with new medium access control architectures. Medium access control is basically the coordination of the nodes in a network for sharing the medium, i.e., the radio in a general case. There are two basic goals. One is to avoid or reduce collisions in the network, since collisions waste network resources such as energy, bandwidth, and computing power but generate no useful outputs. The second goal of medium access control is to maintain high medium utilization. The extreme strategy of collision avoidance would be to let no nodes transmit in the network. In such a case, there would be no collisions in the network, but there is no good throughput either. Therefore, a good medium access control protocol should be able to avoid collisions and at the same maintain high medium utilization. Our one recent project is on improving the IEEE 802.11 Distributed Coordination Functions (DCF). We use a cross-layer approach to improve the capability of the original protocol in avoid collisions and at the same time also reduce the control overhead of the original protocol (i.e., enhance the medium utilization). Our basic idea is to deliver medium access control information through a simple modulation method instead of the traditional bit-based mechanism. The following two figures show some of our evaluation results for the new approach. The first figure shows how the throughput of the network is improved with the new medium access control protocol, and the second figure shows how the medium access delay is reduced with the proposed protocol.